Why does time go forwards, not backwards?
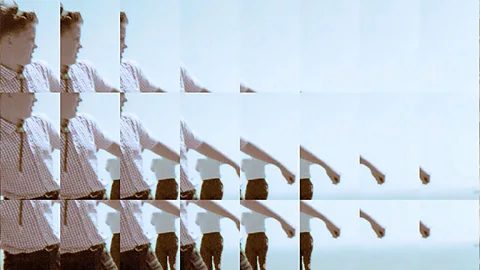
The arrow of time began its journey at the Big Bang, and when the Universe eventually dies there will be no more future and no past. In the meantime, what is it that drives time ever onward?
When Isaac Newton published his famous Principia in 1687, his three elegant laws of motion solved a lot of problems. Without them, we couldn't have landed people on the Moon 282 years later. But these laws brought to physics a new problem, which wasn't fully appreciated until centuries after Newton and still nags at cosmologists today.
The issue is that Newton's laws work about twice as well as we might expect them to. They describe the world we move through every day – the world of people, the hands that move around a clock and even the apocryphal fall of certain apples – but they also account perfectly well for a world in which people walk backwards, clocks tick back afternoon to morning, and fruit soars up from the ground to its tree-branch.
"The interesting feature of Newton's laws, which wasn't appreciated till much later, is that they don't distinguish between the past and the future," says the theoretical physicist and philosopher Sean Carroll, who discusses the nature of time in his latest book The Biggest Ideas in the Universe. "But the directionality to time is its most obvious feature, right? I have photographs of the past, I don't have any photographs of the future."
The problem is not confined to the centuries-old theories of Newton. Virtually all of the cornerstone theories of physics since then have worked just as well going forward in time as they do backwards, says physicist Carlo Rovelli of the Centre for Theoretical Physics in Marseille, France, and the author of books including The Order of Time.
"Starting from Newton, and then Maxwell's theory of electromagnetism, then Einstein's work, and then quantum mechanics, quantum field theory, general relativity, and even quantum gravity – there is no distinction between past and future," Rovelli says. "Which came as a surprise, because the distinction is so evident to all of us. If you make a movie, it's obvious which way is the future and which one is the past."
How does a clear direction of time emerge from these descriptions of the Universe, which all lack their own arrow of time? As Marina Cortês, an astrophysicist at the University of Lisbon, puts it: "There's a lot of implications that start with taking seriously the question, 'Why does time pass?'"
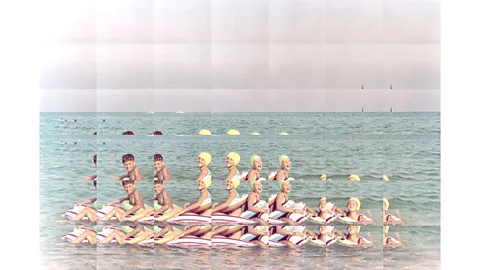
Part of the answer lies at the Big Bang nearly 14 billion years ago. Another insight comes from the opposite extreme, in the Universe's eventual death.
But before embarking on this epic journey back and forth along the timeline of the Universe, it's worth stopping off in 1865, just as the first truly time-directional law of physics came hurtling down the tracks of the Industrial Revolution.
Gathering steam
In the 19th Century, when coal was shovelled into furnaces to generate steam power, scientists and engineers hoping to develop better engines embraced a set of principles that described the relationship between heat, energy and motion. They became known as the laws of thermodynamics.
Entropy and the rise of disorder
When you buy a pack of cards, they tend to be in order – each suit collected neatly together, going from ace to king (or king to ace) in turn. When you give them their first thorough shuffle, what do you expect? Probably, a pack of cards in no discernible order.
Shuffle them a second time – what do you expect now? I bet the answer isn't "the perfectly ordered pack that I originally bought". A better answer might be "a pack of cards in no discernible order, just a different muddle to the one I had before".
One way to think about entropy is as a measure of disorder. The brand new pack of cards has low entropy, and when you shuffle it you increase the entropy, or apparent randomness, of the system.
Similarly, as an object heats up, its entropy rises. In a block of ice, water molecules are closely packed and ordered. As the ice warms and melts into liquid water, the molecules tumble past each other more freely – they are in greater disorder. In steam, the water molecules are more disordered still.
In Germany, 1865, the physicist Rudolf Clausius stated that heat cannot pass from a cold body to a hot one, if nothing else around them changes. Clausius came up with the concept he called "entropy" to measure this behaviour of heat – another way of saying heat never flows from a cold body to a hot one is to say "entropy only ever increases, never decreases" (see box Entropy and the Rise of Disorder).
As Rovelli stresses in The Order of Time, this is the only basic law of physics that can tell apart the past from the future. A ball can roll down a hill or be kicked back to its summit, but heat can't flow from cold to hot.
To illustrate, Rovelli picks up his pen and drops it from one hand to the other. "The reason this stops in my hand is that it has some energy, and then the energy is turned into heat and it warms up my hand. And the friction stops the bouncing. Otherwise, if there was no heat, this would bounce forever, and I would not distinguish the past from the future."
So far, so straightforward. That is, until you start to consider what heat is on a molecular level. The difference between hot things and cold things is how agitated their molecules are – in a hot steam engine, water molecules are very excited, careening around and colliding into each other rapidly. The very same water molecules are less agitated when they coalesce as condensation on a windowpane.
Here's the problem: when you zoom in to the level of, say, one water molecule colliding and bouncing off another, the arrow of time disappears. If you watched a microscopic video of that collision and then you rewound it, it wouldn’t be obvious which way was forwards and which backwards. At the very smallest scale, the phenomenon that produces heat – collisions of molecules – is time-symmetric.
This means that the arrow of time from past to future only emerges when you take a step back from the microscopic world to the macroscopic – something first appreciated by the Austrian physicist-philosopher Ludwig Boltzmann.
"So the direction of time comes from the fact that we look at big things, we don't look at the details," says Rovelli. "From this step, from the fundamental microscopic vision of the world to the coarse-grained, the approximate description of the macroscopic world – this is where the direction of time comes in.
"It's not that the world is fundamentally oriented in space and time," Rovelli says. It's that when we look around, we see a direction in which medium-sized, everyday things have more entropy – the ripened apple fallen from the tree, the shuffled pack of cards.
While entropy does seem to be inextricably bound up with the arrow of time, it feels a bit surprising – perhaps even disconcerting – that the one law of physics that has a strong directionality of time built into it loses this directionality when you look at very small things.
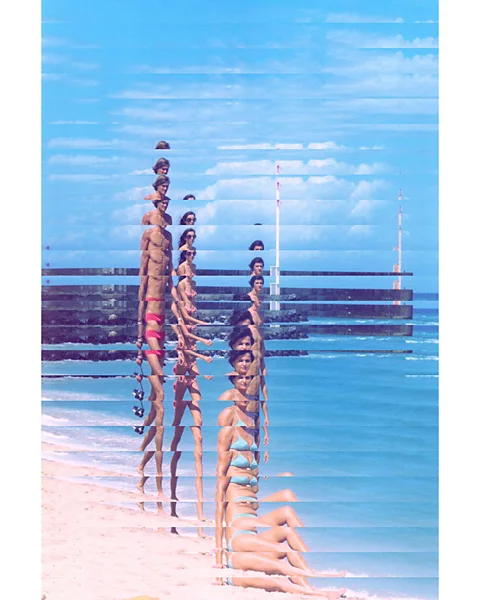
"What is entropy?" Rovelli says. "Entropy is simply how much we're forgetting about the microphysics, how much we are forgetting about the molecules."
The beginning and the end
If there is an arrow of time, where did it come from in the first place?
"The answer is embedded in the beginning of the Universe," says Carroll. "The answer is because the Big Bang had low entropy. And still, 14 billion years later we are swimming in the aftermath of that tsunami that started near the Big Bang. That's why time has a direction for us."
The extraordinarily low entropy of the Universe at the Big Bang is both an answer and an enormous question. "The thing we understand the least about the nature of time, is why the Big Bang had low entropy, why the early Universe was like that," says Carroll. "And I think honestly, as a working cosmologist, I think that my fellow cosmologists have dropped the ball on this one. They don't really take that problem seriously enough."
Carroll published a paper in 2004 with his colleague Jennifer Chen, in which they aimed to explain why the Universe had such low entropy close to the Big Bang, rather than just assuming or accepting this was the case. "There's plenty of loopholes in the theory, plenty of aspects of it that are not completely baked – but I also think it is by far the best theory on the market," says Carroll. "It doesn't cheat."
Other cosmologists agree that it is indeed time to turn serious thought to this problem of the Universe's low entropy origins. "The likelihood of our current Universe having initial conditions of this kind, and not any other kind, is around one in 10 to the 10 to 124 (1:10^10^124)," says Cortês. (Another way of saying it is that the event had a probability of 0.00…01 – with 10^(10^124) zeroes omitted – a number so large it's awkward to express in conventional maths, Cortês notes.) "I mean I could safely say, this is the largest number in modern physics, outside of philosophy or mathematics."
Simply taking such unlikely low-entropy origins as given is a grand case of "shoving the problem under the rug", Cortês says. "If physicists keep doing this, after a while it's going to be a very big pile under the rug. It's left to us cosmologists to explain why time only moves forward."
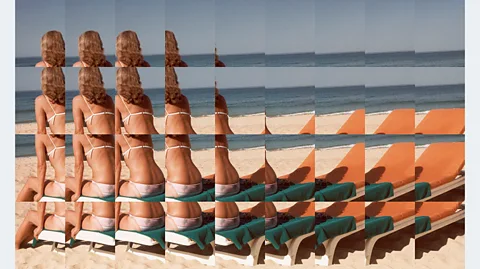
Even if we don't yet know why, the Universe's low entropy past is a plausible source of time's arrow. Like most things that have a beginning, the arrow will also have an end. The first person to spot this was, once again, the Austrian physicist Ludwig Boltzmann.
"Boltzmann thought, 'ah, entropy is growing in the Universe and maybe it's going to maximum at some point'," says Rovelli. At that point, heat would be evenly distributed throughout the Universe, no longer flowing from one place to another.
How will the Universe end?
Heat death is not the only way the Universe as we know it could meet its end.
Several other options have been proposed, among them the Big Bounce and other cyclical models. But heat death is by far the leading contender, says cosmologist Sean Carroll.
"It's not in any sense established, but it's just the easiest extrapolation from what we know, because you don't have to work that hard to get there," says Carroll. All the Universe has to do to get to heat death, is carry on exactly what it's doing indefinitely. "There's no reason for it to ever stop."
Read more about how the Universe might end.
There would be no energy available in a useful form for doing work – in other words, almost nothing interesting would be happening throughout the entire Universe. As astrophysicist Katie Mack describes it, "As that process continues, everything is decaying so much that all that’s left is the waste heat of everything that ever existed in the Universe." This fate is known as the thermal death of the Universe, or heat death.
"Stars will stop burning, nothing will happen anymore. There will be nothing but small thermal fluctuations," says Rovelli. "Suppose this happens – and we don't know for certain if it's going to happen, but suppose it does – should we say that there is no time direction there? Of course there's no time direction, because every phenomenon that happened one way could also go one way or the other. Nothing will distinguish the two directions of time."
This is perhaps the strangest thing about the arrow of time: "It only lasts for a little while," says Carroll.
It's very hard to picture what might happen if the arrow of time eventually vanishes. "When we think we produce heat in our neurons," says Rovelli. "Thinking is a process in which the neuron needs entropy to work. Our sense of time passing is just what entropy does to our brain."
The arrow of time that arises from entropy brings us a long way closer to understanding why time only goes forward. But there may be more arrows of time than this one – in fact there is arguably an entire volley of arrows of time pointing from the past to the future. To understand these, we have to step from physics into philosophy.
Human time
The ways that we intuitively understand and experience time shouldn't be taken lightly, says Jenann Ismael, professor of philosophy at Columbia University, New York. If you think about your own experience of time, you may soon be able to recognise several of the psychological arrows that form a core part of human experience. One of these arrows is what Ismael terms "flow".
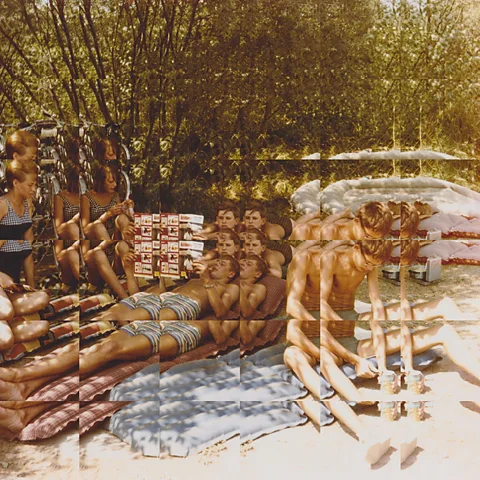
"If you look out at the world, you don't experience a purely static representation of the instantaneous state of the world," she says, like in a movie made up of a number of static frames every second. "We see directly that the world is changing."
This experience of the flow of time is built into our perception. "Vision isn't like a movie camera at all," says Ismael. "Actually what happens is your brain is collecting information over some temporal period. It's integrating that information so that at any given moment, what you're seeing is a computation that the brain has done. So that you not only see that things are moving, you see how fast they're moving, the direction in which they're moving. So the whole time, your brain is integrating information over temporal intervals and giving you the result. So you see time, in a way."
There's a second feature of time that Ismael distinguishes from flow, which she terms "passage".
The idea of passage is closely bound up with time-oriented experiences such as memory and anticipation. Take the example of a wedding, or any much-anticipated life event. Our experience of these moments has many layers – from the fractious planning stages, to the intensity of the day itself, to recollections that stay with us for years. There is a directionality to these different experiences: the way we anticipate an event in the future is fundamentally different from how we remember it when it's passed.
"All of that is part of what I think of as the experience of passage, this idea that we experience every event as anticipated from the past, experienced in the present, remembered in retrospect," says Ismael. "It's kind of Proustian in its density."
These aspects of the directionality of psychological time – as well as many others, like the sense of openness we have about the future but not the past – could all trace their roots back to the arrow of time born of the Industrial Revolution.
"I think it does all come back to entropy," says Ismael. "I see no reason now to think that the kinds of arrows that are involved in human psychology are anything but ultimately rooted in the entropic arrow. But it's an empirical question. This project to understand human experience in relation to the entropic arrow, I've no reason to think it's going to fail."
That project is what Carroll hopes to do, taking several features of our experience of time and relating them back to entropy. His first target is causality, another element of the arrow of time, as causes happen before their effects.
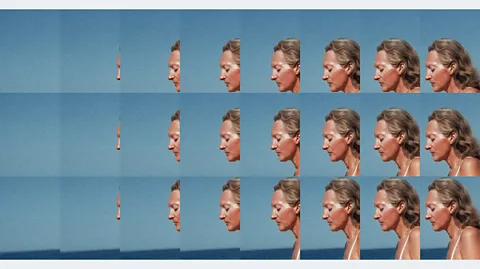
To say the least, this project is a major undertaking for all physicists and philosophers involved. And still, lurking in the shadows behind all such efforts, there remains that nagging question about why entropy was so low in the earliest Universe.
"I think we understand why we have this sense of flowing," says Rovelli. "We understand why the past seems fixed to us that the future seems open. We understand why there are irreversible phenomena, and we can reduce all that to the second law of thermodynamics, to the rise of entropy.
"It's very much related to the fact that if we trace it back, back, back, to fact that the Universe started very small, in a very peculiar situation. Then somehow, it's falling down from that peculiar situation.
"But of course there's one question open, I mean, why? Why did it start in that particular way?"
--
Images from Edouard Taufenbach, Spéculaire.
--
* Martha Henriques is Editor of BBC Future Planet, and tweets at @Martha_Rosamund
--